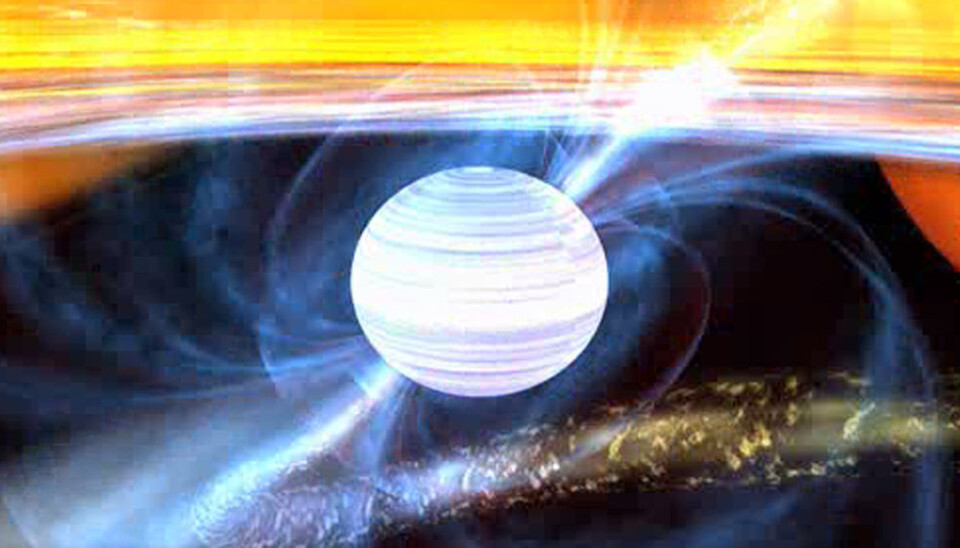
Neutron star riddle solved
New theoretical calculations show how quickly rotating neutron stars – millisecond pulsars – slow down when they no longer attract matter from their companion stars.
Astrophysicists studying millisecond pulsars, which devour stars they are paired with, have long been puzzled by a slow down in the millisecond pulsars’ rotation once its meal is over.
In astrophysics, a millisecond pulsar – a very quickly rotating neutron star – is usually paired with another star, called a companion star. And the millisecond pulsar often attracts matter from its companion star, devouring it in the process.
The millisecond pulsar uses its enormous gravitational field to suck up gasses, and gain angular momentum, from the companion star’s surface until the companion star has been reduced to a white dwarf – a very dense, small star.
This accretion process gives the millisecond pulsar new life and energy, allowing it to rotate around its own axis very quickly – just as ice-skaters can pirouette faster by pulling their arms close to their bodies.
Rotation slows after accretion
Accretion and the accompanying high-speed rotation are well known to astrophysicists, but no-one has described the situation immediately after the millisecond pulsar no longer attracts matter from its companion star.
By combining existing theories for the way stars develop with calculations of the forces affecting millisecond pulsars, the Danish astrophysicist Thomas Tauris – currently a guest professor at the stellar physics group of the Argelander Institute for Astronomy at the University of Bonn in Germany – has successfully developed a computer model for a millisecond pulsar’s behaviour when the accretion process stops.
The very quickly rotating neutron star, the millisecond pulsar, has a built-in brake – its expanding magnetosphere (an envelope of magnetically confined, charged particles around the millisecond pulsar) – that works automatically when accretion stops.
“I’ve been looking at what happens the moment that the neutron star no longer sucks gases from its companion – something nobody has apparently tried to calculate before,” says Tauris. “It was only after I’d done it that I realised how important my results are, as they can help solve a number of riddles.”
Recent discovery
Millisecond pulsars are a relatively recent discovery – they were first discovered in 1982 – and only 200 of these neutron stars are known.
It is thought that millisecond pulsars were originally created from old neutron stars with an extremely high mass density and strong magnetic fields; neutron stars form as the inner core of a heavy star explodes – an event called a supernova explosion.
Although neutron stars will die out in time, those that are located close to another star can extend their life by attracting matter and rotational energy from their companion star.
As the neutron star attracts this energy, it rotates faster and faster around its own axis until it reaches a speed of 100 to 700 rotations per second – so it rotates once in every 1-10 ms (milliseconds – hence its name).
All the time the millisecond pulsar is accreting from the companion star, it emits a pulse of high-energy X-rays from its magnetic poles for every rotation.
As soon as the companion star’s surface has been stripped of usable matter, the millisecond pulsar’s X-ray emissions are replaced by radio waves of a lower energy, and apparently at a slightly reduced tempo.
The star has turned from an X-ray millisecond pulsar to a radio millisecond pulsar.
The transition has been revealed through observations, but the physical processes behind the transition have been puzzling. Now, however, Tauris’s model has thrown light on the process.
Slowing down
As the matter available on the companion star runs out, less and less gas flows to the rotating millisecond pulsar, and this reduces the pressure on the millisecond pulsar’s magnetosphere, which can now expand and start to act as a barrier.
The magnetosphere barrier rotates at the same speed as the millisecond pulsar, and the gas and other matter from the companion star, which can no longer pass through the magnetosphere, are spread in all directions.
As a result, because forces acting on a body must be balanced, the millisecond pulsar loses rotational momentum and starts to rotate at a marginally slower rate.
At the same time, the actual expansion of the magnetosphere has a slowing effect on the millisecond pulsar. But the speed of the magnetosphere’s expansion is higher than the speed at which its slowing effect is transmitted to the millisecond pulsar’s core. As a result, the millisecond pulsar only loses little more than half its rotational speed, with a consequent increase in rotation time, so it can still be called a millisecond pulsar.
Observations show that the average rotational period for X-ray millisecond pulsars is 3.3 ms, and 5.5 ms for radio millisecond pulsars.
“The very same process responsible for spinning up old neutron stars to extraordinary fast spin rates with periods of 1-10 ms is actually also causing the millisecond pulsars to spin down again,” says Tauris.
“This knowledge has thrown new light on the formation of radio millisecond pulsars. Whether the process accelerates or brakes the millisecond pulsar spin depends on the size of the magnetosphere and the rotational period of the millisecond pulsar.”
Age problem
Astronomers have always tried to calculate the age of neutron stars, as age is very important for understanding the origin and development of these extreme objects.
The only source of age information used so far has been the time between millisecond pulsars’ X-ray or radio pulses and the deceleration speed of the millisecond pulsars’ rotation, although this rotation speed has not been known precisely. This gives the characteristic spin-down age of the star.
“Another interesting result of my findings is that the so-called characteristic spin-down age of radio millisecond pulsars has been shown to be absolutely untrustworthy,” says Tauris.
“I show how a radio millisecond pulsar can appear to have a characteristic age exceeding the age of the Universe, despite just having been recycled. The only reliable age estimator for a radio millisecond pulsar, although still somewhat uncertain, is the cooling age of its white dwarf companion (the remnant of the former companion star responsible for spinning-up the pulsar).”
Testing Einstein’s Theory of Relativity
Millisecond pulsars are very dense stars and they have extremely strong magnetic fields – up to a million times stronger than the fields so far generated in a laboratory.
“Studying millisecond pulsars and other forms of neutron stars allows scientists to gain knowledge that they cannot investigate on Earth,” says the researcher.
Of special interest is millisecond pulsars’ precision and stability as timepieces, so scientists can carry out unprecedented tests of gravitational theories in strong magnetic fields, of Einstein’s theory of four-dimensional space-time, and study what space looks like around a millisecond pulsars’ companion star.
Together with his colleagues in Bonn, Tauris is considering studying how millisecond pulsars behave in the vicinity of a black hole, the most extreme object of all. To date, millisecond pulsars have only been observed in orbit with white dwarves or other neutron stars.
“We would very, very much like to find a millisecond pulsar near a black hole, so we can test and compare various gravitational theories,” says Tauris. “That’s one of the great challenges in astronomy at the moment.”
--------------------------------------------------
Read this article in Danish at videnskab.dk
Translated by: Michael de Laine
Scientific links
External links
- Thomas Tauris' profile
- About millisecond pulsars (Wikipedia)
- About millisecond pulsars (NASA, animation)