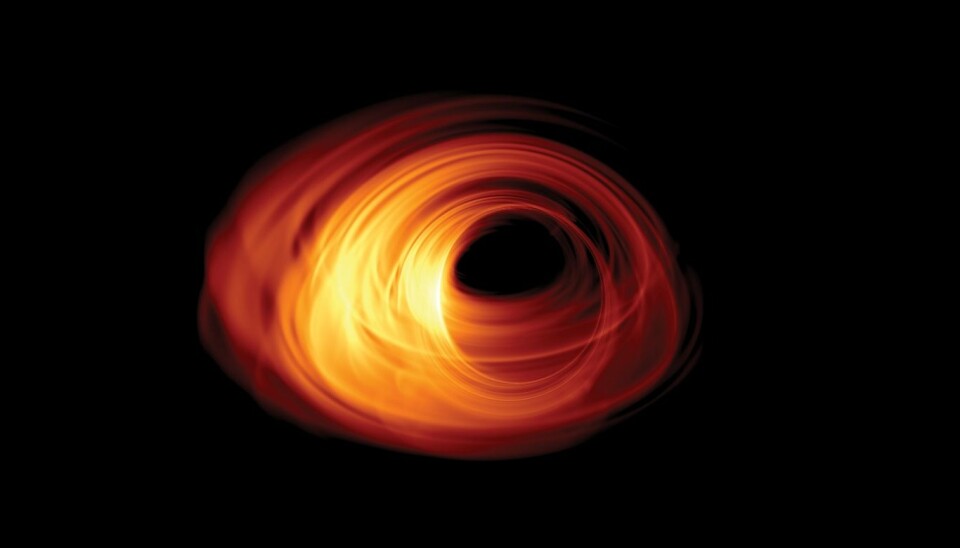
Will we ever see a black hole?
In the shadowy regions of black holes two fundamental theories describing our world collide. Can these problems be resolved and do black holes really exist? First, we may have to see one and scientists are trying to do just this.
Of all the forces in physics there is one that we still do not understand at all: Gravity.
Gravity is where fundamental physics and astronomy meet, and where the two most fundamental theories describing our world - quantum theory and Einstein’s theory of spacetime and gravity (aka. the theory of general relativity) – clash head on.
The two theories are seemingly incompatible. And for the most part this isn’t a problem. They both live in distinct worlds, where quantum physics describes the very small, and general relativity describes the very largest scales.
Only when you get to very small scales and extreme gravity, do the two theories collide, and somehow, one of them gets it wrong. At least in theory.
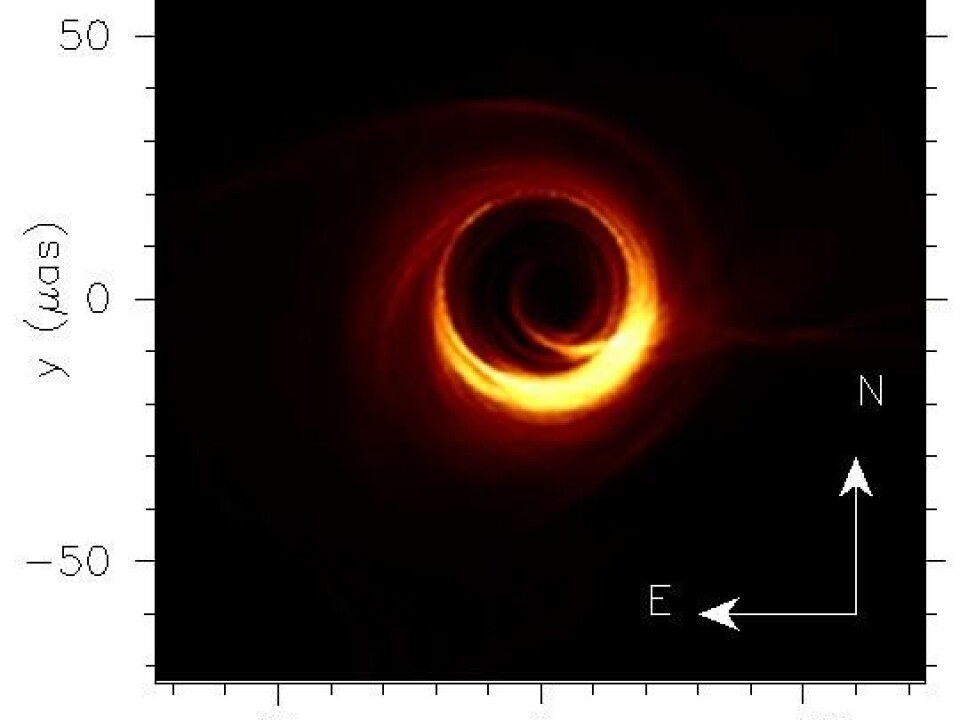
But there is once place in the universe where we could actually witness this problem occurring in real life and perhaps even solve it: the edge of a black hole. Here, we find the most extreme gravity. There’s just one issue – nobody has ever actually ‘seen’ a black hole.
Read More: Gravity: it is all in your head
So, what is a black hole?
Imagine that the entire drama of the physical world unfolds in the theatre of spacetime, but gravity is the only ‘force’ that actually modifies the theatre in which it plays.
The force of gravity rules the universe, but it may not even be a force in the traditional sense. Einstein described it as a consequence of the deformation of spacetime. And perhaps it simply does not fit the standard model of particle physics.
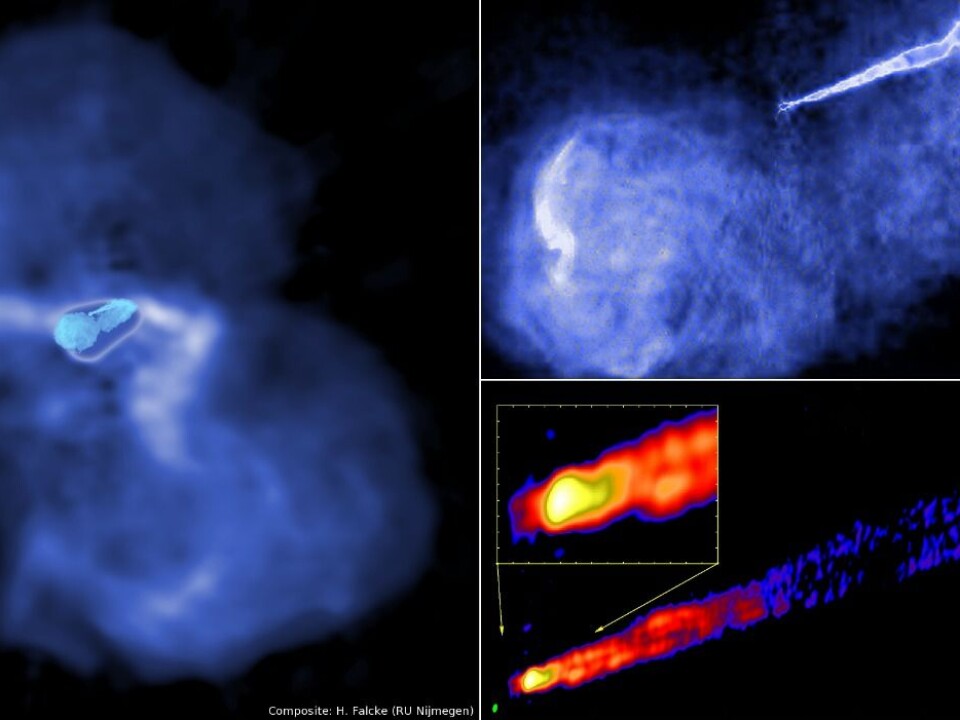
When a very big star explodes at the end of its lifetime, its innermost part will collapse under its own gravity, since there is no longer enough fuel to sustain the pressure working against the force of gravity (yes, gravity feels like a force after all, doesn’t it!).
The matter collapses and no force in nature is known to be able to stop that collapse, ever.
In an infinite time, the star will have collapsed into an infinitely small point: a singularity – or to give it another name, a black hole.
Of course, in a finite time the stellar core will have collapsed into something of a finite size and this would still be a huge amount of mass in an insanely small region and it still is called a black hole!
Black holes do not suck in everything around them
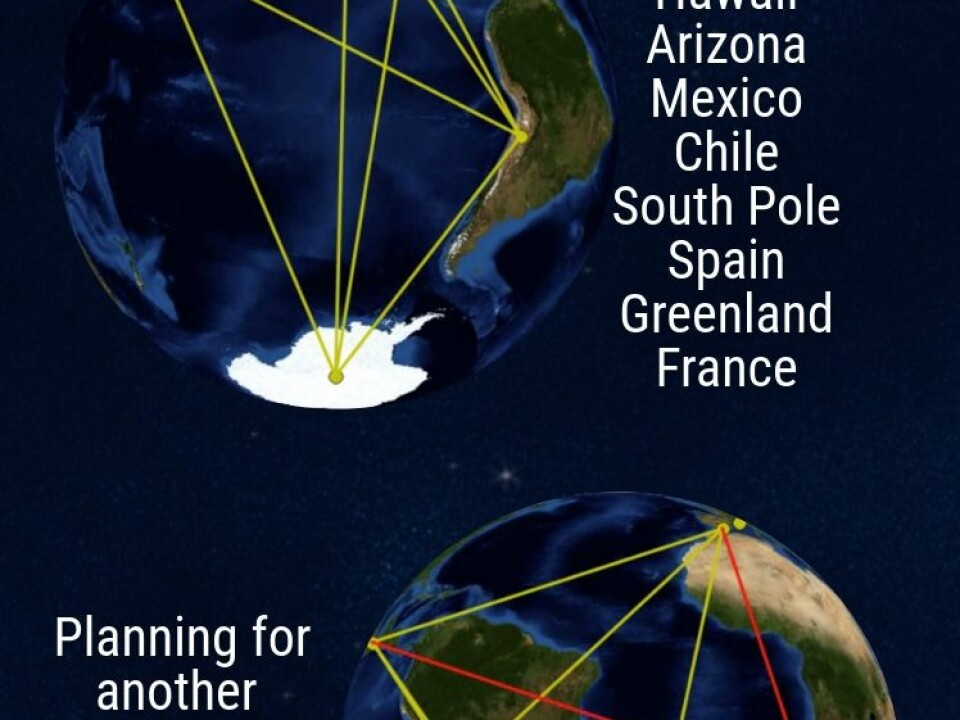
Interestingly, it is not true that a black hole will inevitably draw everything in.
In fact, whether you are orbiting a star or a black hole that formed from a star, it does not make a difference, so long as the mass is the same. The good old centrifugal force and your angular momentum will keep you safe and stop you from falling in.
Only when you fire your giant rocket thrusters to brake your rotation, will you start falling inwards.
However, once you fall towards a black hole you will be accelerated to higher and higher speeds, until you eventually reach the speed of light.
Read More: Stephen Hawking: Master of the Multiverse
Why are quantum theory and general relativity incompatible?
At this point everything goes wrong as, according to general relativity, nothing should move faster than the speed of light.
Light is the substrate used in the quantum world to exchange forces and to transport information in the macro world. Light determines how fast you can connect cause and consequences.
If you go faster than light, you could see events and change things before they happen. This has two consequences:
- At the point where you reach the speed of light while falling inwards, you would also need to fly out at the speed of light to escape that point, which seems impossible. Hence, conventional physical wisdom will tell you that nothing can escape a black hole, once it has passed that point, which we call the “event horizon.”
- It also means that suddenly basic principles of quantum information preservation are brutally violated – conserved quantum quantities can simply disappear behind a wall of silence.
Whether that is true and whether and how the theory of gravity (or of quantum physics) needs to be modified is a question of intense debate among physicists, and none of us can say which way the argument will lead in the end.
Do black holes even exist?
Of course, all this excitement would only be justified, if black holes really existed in this universe. So, do they?
In the last century strong evidence has mounted that certain binary stars with intense X-ray emissions are in fact stars collapsed into black holes.
Moreover, in the centres of galaxies we often find evidence for huge, dark concentrations of mass. These might be supermassive versions of black holes, possibly formed through the merger of many stars and gas clouds that have sunk into the centre of a galaxy.
The evidence is convincing, but circumstantial. At least gravitational waves have let us ‘hear’ the merger of black holes, but the signature of the event horizon is still elusive and so far, we have never actually ‘seen’ a black hole – they simply tend to be too small and too far and, in most cases, yes, black...
Read More: Gravitational-wave astronomy will change our understanding of the universe
So, what would a black hole actually look like?
If you could look straight into a black hole you would see the darkest dark, you can imagine.
But, the immediate surroundings of a black hole could be bright as gasses spiral inwards –slowed down by the drag of magnetic fields they carry along.
Due to the magnetic friction the gas will heat up to enormous temperatures of up to several tens of billion degrees and start to radiate UV-light and X-rays.
Ultra-hot electrons interacting with the magnetic field in the gas will start producing intense radio emission. Thus, black holes can glow and could be surrounded by a ring of fire that radiates at many different wavelengths.
A ring of fire with a dark, dark centre
In their very centre, however, the event horizon still lurks and like a bird of prey it catches every photon that gets too close.
Since space is bent by the enormous mass of a black hole, light paths will also be bent and even form into almost concentric circles around the black hole, like serpentines around a deep valley. This effect of circling light was calculated already in 1916 by the famous Mathematician David Hilbert only a few months after Albert Einstein finalised his theory of general relativity.
After orbiting the black hole multiple times, some of the light rays might escape while others will end up in the event horizon. Along this complicated light path, you can literally look into the black hole. The nothingness you see is the event horizon.
If you were to take a photo of a black hole, what you would see would be akin to a dark shadow in the middle of a glowing fog of light. Hence, we called this feature the shadow of a black hole .
Interestingly, the shadow appears larger than you might expect by simply taking the diameter of the event horizon. The reason is simply, that the black hole acts as a giant lens, amplifying itself.
Surrounding the shadow will be a thin ‘photon ring’ due to light circling the black hole almost forever. Further out, you would see more rings of light that arise from near the event horizon, but tend to be concentrated around the black hole shadow due to the lensing effect.
Read More: Q&A: Professor Brian Cox talks scicomm
Fantasy or reality?
Is this pure fantasy that can only be simulated in a computer? Or can it actually be seen in practice? The answer is that it probably can.
There are two relatively nearby supermassive black holes in the universe which are so large and close, that their shadows could be resolved with modern technology.
These are the black holes in the center of our own Milky Way at a distance of 26,000 lightyears with a mass of 4 million times the mass of the sun, and the black hole in the giant elliptical galaxy M87 (Messier 87) with a mass of 3 to 6 billion solar masses.
M87 is a thousand times further away, but also a thousand times more massive and a thousand times larger, so that both objects are expected to have roughly the same shadow diameter projected onto the sky.
Like seeing a grain of mustard in New York from Europe
Coincidentally, simple theories of radiation also predict that for both objects the emission generated near the event horizon would be emitted at the same radio frequencies of 230 GHz and above.
Most of us come across these frequencies only when we have to pass through a modern airport scanner but some black holes are continuously bathed in them.
The radiation has a very short wavelength of about one millimetre and is easily absorbed by water. For a telescope to observe cosmic millimetre waves it will therefore have to be placed high up, on a dry mountain, to avoid absorption of the radiation in the Earth’s troposphere.
Effectively, you need a millimetre-wave telescope that can see an object the size of a mustard seed in New York from as far away as Nijmegen in the Netherlands. That is a telescope a thousand times sharper than the Hubble Space Telescope and for millimetre-waves this requires a telescope the size of the Atlantic Ocean or larger.
Soon@M87 workshop: My simulation of the relativistic jet from the supermassive black hole in the core of M87 galaxy. pic.twitter.com/PCJZKRuyUP
— Monika Moscibrodzka (@mmosc_m) May 20, 2016
A virtual Earth-sized telescope
Fortunately, we do not need to cover the Earth with a single radio dish, but we can build a virtual telescope with the same resolution by combining data from telescopes on different mountains across the Earth.
The technique is called Earth rotation synthesis and very long baseline interferometry (VLBI). The idea is old and has been tested for decades already, but it is only now possible at high radio frequencies.
The first successful experiments have already shown that event horizon structures can be probed at these frequencies. Now high-bandwidth digital equipment and large telescopes are available to do this experiment on a large scale.
Read More: New Greenland telescope is up and running
Work is already underway
I am one of the three Principal Investigators of the BlackHoleCam project. BlackHoleCam is an EU-funded project to finally image, measure and understand astrophysical black holes. Our European project is part of a global collaboration known as the Event Horizon Telescope consortium – a collaboration of over 200 scientists from Europe, the Americas, Asia, and Africa. Together we want to take the first picture of a black hole.
In April 2017 we observed the Galactic Center and M87 with eight telescopes on six different mountains in Spain, Arizona, Hawaii, Mexico, Chile, and the South Pole.
All telescopes were equipped with precise atomic clocks to accurately synchronise their data. We recorded multiple petabytes of raw data, thanks to surprisingly good weather conditions around the globe at the time.
We are all excited about working with this data. Of course, even in the best of all cases, the images will never look as pretty as the computer simulations. But, at least they will be real and whatever we see will be interesting in its own right.
To get even better images telescopes in Greenland and France are being added. Moreover, we have started raising funds for additional telescopes in Africa and perhaps elsewhere and we are even thinking about telescopes in space.
A ‘photo’ of a black hole
If we actually succeed in seeing an event horizon, we will know that the problems we have in rhyming quantum theory and general relativity are not abstract problems, but are very real. And we can point to them in the very real shadowy regions of black holes in a clearly marked region of our universe.
This is perhaps also the place where these problems will eventually be solved.
We could do this by obtaining sharper images of the shadow, or maybe by tracing stars and pulsars as they orbit around black holes, through measuring spacetime ripples as black holes merge, or as is most likely, by using all of the techniques that we now have, together, to probe black holes.
Read More: The Big Bang – an eyewitness account
A once exotic concept is now a real working laboratory
As a student, I wondered what to study: particle physics or astrophysics? After reading many popular science articles, my impression was that particle physics had already reached its peak. This field had established an impressive standard model and was able to explain most of the forces and the particles governing our world.
Astronomy though, had just started to explore the depths of a fascinating universe. There was still a lot to be discovered. And I wanted to discover something.
In the end, I chose astrophysics as I wanted to understand gravity. And since you find the most extreme gravity near black holes, I decided to stay as close to them as possible.
Today, what used to be an exotic concept when I started my studies, promises to become a very real and very much visible physics laboratory in the not too distant future.
---------------