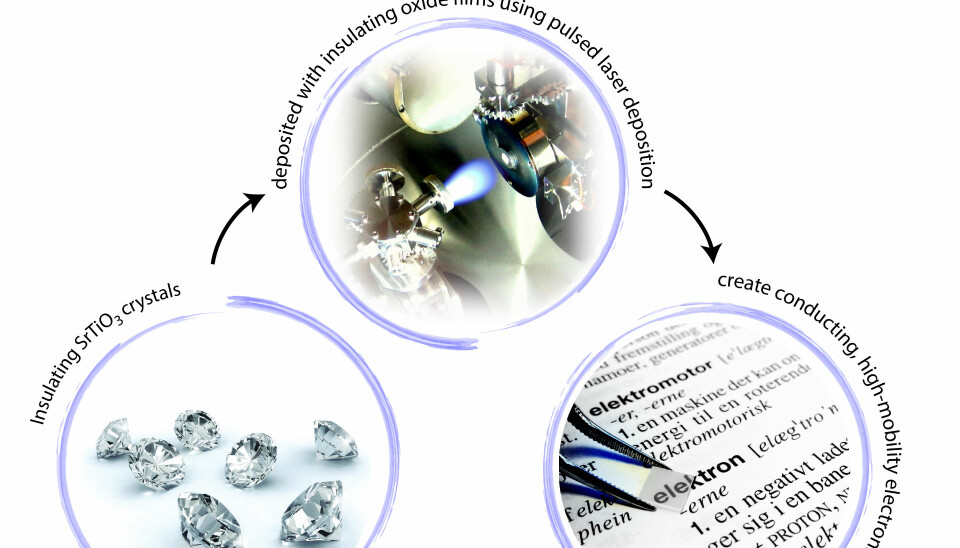
When insulators become electronics
A new, superconducting oxide system multiplies the electron mobility in electronic oxide transistors. Benefits include superconducting nanotransistors, self-charging electronic devices and a new type of RAM.
As part of a research group at the Department of Energy Conversion and Storage at the Technical University of Denmark (DTU), we have assisted in the discovery of a new superconducting oxide system.
This discovery paves the way for using oxide structures in electronic circuits that not only contain high-mobile electrons – i.e. where the electrons move rapidly in the circuits – but also have room for 100 times more components per square centimetre compared to existing electronic chips.
The new oxide system also opens up for creating a new type of memory for computers (resistive RAM), superconductive nanotransistors and self-charging electric circuits. These circuits can be charged by e.g. converting body heat into electricity, so that the battery in a wristwatch or a mobile phone never runs out of power.
Astounding results
Our discovery is inspired by the Nobel Prize speech of physicist Herbert Kroemer in 2000, where he said: ”Often, it may be said that the interface is the device.”
Kroemer referred to the situation where two materials are placed in intimate contact. Here, extraordinary phenomena can arise at the interface between the materials – phenomena that are not present in any of the two materials, but only at the interface.
The concept has been known for millennia and is used in countless areas. It is, for instance, used extensively in modern electronics to create transistors, which are the cornerstone of all computers.
However, with the oxides we are pouring new wine into old bottles in the hunt for new electronic components by growing a thin oxide layer on top of another oxide, strontium titanate (SrTiO3).
The result is astounding: even though the two oxides are electrically insulating and non-magnetic, the interface becomes conducting and magnetic.
If it is cooled down, the interface becomes superconducting, which means that unlike regular cables, the interface can conduct electricity without any loss of energy.
Heat converted into electricity
In addition, an electrical potential can be applied, which increases the conductivity 10,000 times at room temperature, while the superconductivity can be turned on and off at low temperatures, thus making superconducting transistors.
If the electrical potential is applied through a very sharp needle, it is possible to draw electrical circuits (normal or superconducting) with dimensions down to only a few nanometres.
If surplus heat is provided, part of this heat can be converted into electricity through the thermoelectric effect.
Oxide systems are the solution
The palette of interface phenomena continues – and it all takes place within a single system. The colourful palette arises as a result of the unique electron structure found in oxides and thus does not occur in the conventional semiconductor materials that are used in modern electronics.
The application of oxides is, however, limited by the mobility of the electrons in the interface: the electron mobility is simply too low for many high-tech applications.
And it is here that we, as part of Professor Nini Pryds’ research group at DTU, assisted in taking a big step towards solving this challenge. Our group discovered a new oxide system, which was described in the journal Nature Communications earlier this year.
In this system, the electron mobility is more than ten times higher than in typical competing oxide systems.
Atomic precision forms very few defects
The key to the very high electron mobility was found by growing aluminium (III) oxide (Al2O3) on top of SrTiO3 with atomic precision using an advanced technique known as pulsed laser deposition.
Al2O3 ’fits’ so well with SrTiO3 that the quality of the interface becomes so high that only few defects are formed. Without hitting the defects, the electrons can move faster at the interface, and this is how the high mobility is obtained.
However, the development does not stop here, as we are continuing to increase the mobility, while also finalising the characterisation of a new high-mobility oxide system.
--------------------
Read the Danish version of this article at videnskab.dk
Translated by: Dann Vinther