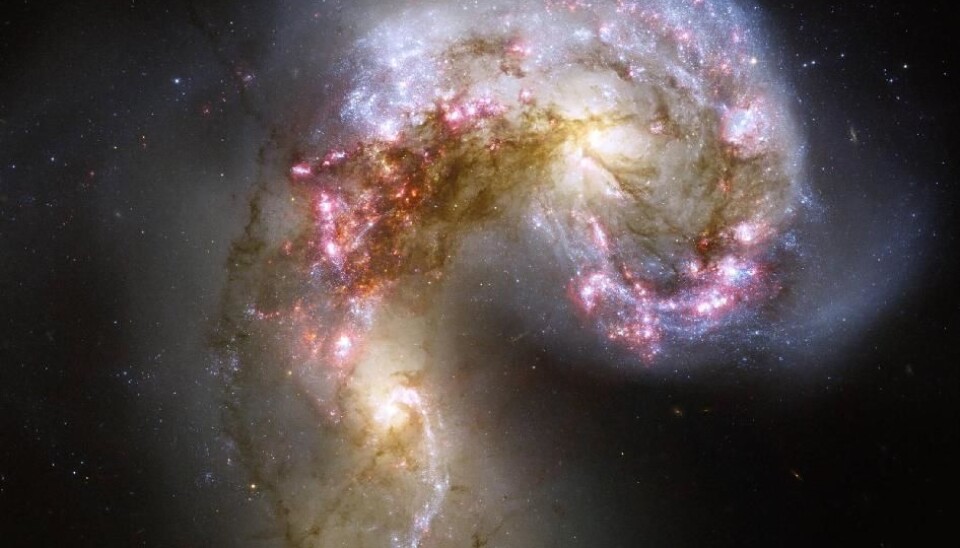
Neutrinos’ mass found with help of galaxies
The mass of the neutrino, the lightest particle in the universe, has now been computed more precisely than before.
Neutrinos have long been seen as the lightest particles in the universe – particles that move close to the speed of light. But their precise mass has been a mystery for almost as long.
Now an international team of scientists under Danish leadership has managed to shed more light on the matter.
After studying galaxies for more than 200 nights, and following thousands of calculations, the cosmologists have found a new way of computing the mass of the neutrino. The method gives a far more precise result than previously used methods.
The new findings have just been published in the scientific journal Physical Review D Rapid Communication.
When neutrinos leave a galaxy they remove mass from the galaxy and change its gravitational potential. Analysing the structure of the galaxies makes it possible to determine the mass of the neutrinos.
Signe Riemer-Sørensen
”Our studies show that it’s possible to measure the mass of neutrinos with far greater accuracy using distant galaxies than through physics experiments in a laboratory,” says Signe Riemer-Sørensen, a Danish post-doc at the School of Mathematics and Physics at the University of Queensland in Australia, who led the studies.
Escaping neutrinos give lighter galaxies
Riemer-Sørensen and her colleagues from the US, South Africa and Canada estimate the neutrino’s mass to lie between 0.29 electron volts (eV) and 0.60 eV.
In comparison, an electron has a mass of about 0.511 MeV, up to two million times heavier.
Neutrinos are the smallest particles we know. But they nevertheless had an impact on the way the universe and its galaxies were formed out of the mass of the other matter floating around the cosmos after the Big Bang billions of years ago.
In the early stages of the universe, neutrinos pushed against the other matter.
Light neutrinos would not have distributed this other matter very well. But heavier neutrinos would have spread this matter far more widely, which would have made it more difficult for galaxies to form. So the mass of the neutrinos was decisive for determining how many galaxies there are today, and how close together they are.
The number of galaxies and their density are indicators of the mass of the particles that were instrumental in deciding their number and distribution.
Neutrinos can move the other matter around precisely because neutrinos move through the universe almost at the speed of light. Such particles are able to escape from large structures like galaxies, even though these structures contain a lot of dark matter and their enormous gravitational fields try to constrain the neutrinos.
”When neutrinos leave a galaxy they remove mass from the galaxy and change its gravitational potential,” says Riemer-Sørensen. ”Analysing the structure of the galaxies makes it possible to determine the mass of the neutrinos.”
Model for galaxy distribution not good enough
For making such analyses, the scientists need a good theoretical description of the distribution of galaxies in space.
Initially, Riemer-Sørensen and her colleagues tested the models for the distribution of the galaxies that had previously been used in similar studies. The results showed that these old models were simply not good enough for use in determining neutrinos’ mass more precisely.
Instead, the scientists chose another path – adapting theoretical models to computer simulations of galaxy structures and their development. This worked and gave the scientists a far better result.
Studied thousands of galaxies
As a starting point, Riemer-Sørensen and her colleagues used data from the WiggleZ Dark Energy Survey, a massive three-dimensional map of 240,000 very distant, blue-light galaxies.
Completed last year, this was said to be the first individual galaxy survey to span seven billion years back in time – more than halfway back to the Big Bang.
There is a very strong correlation between the distribution of these galaxies and the underlying dark matter. Thus they form a good foundation when determining how much mass the galaxy has lost due to escaping neutrinos.
The precise location of the many galaxies in this survey reveals information about the mass of the neutrinos.
”Heavier red-light galaxies were used in previous analyses,” says Riemer-Sørensen. “But the problem with that approach is that they are often found in the centre of galactic clusters, making it difficult to measure the dark matter precisely.”
New study outshines lab tests
Advanced laboratory experiments have previously been used for determining the mass of neutrinos. These experiments have successfully measured the difference in mass between different neutrino types. But such differences are of limited use when scientists do not know the absolute mass of neutrinos – which is what the new study can reveal.
The new method – cosmological galaxy measurements based on the WiggleZ Dark Energy Survey – is thus more effective than laboratory experiments on Earth.
Although the new method is good, there is room for improvement, and the research team is refining their neutrino mass measurements by combining their results with other independent data sets, such as measurements from other astronomical observations.
Neutrinos’ mass can help solve other mysteries
The scientists look forward to a precise measure of neutrinos’ mass, as this will help them solve numerous mysteries and paradoxes in their branch of physics.
Today, neutrinos are considered as being without mass in the ‘standard model’ for particle physics, which describes how all the matter and energy around us is made up of a limited number of elementary particles.
”This means that there is something fundamentally wrong with the ‘standard model’ for particle physics, or there are things missing from it,” says Riemer-Sørensen.
”The ‘standard model’ has otherwise very successfully predicted various phenomena in particle physics.”
Better telescopes on the horizon
Apart from a better understanding of the smallest building block of matter, a precise measure of neutrinos’ mass will also make more precise particle accelerator detectors possible.
In addition, it will lead to greater knowledge of the processes in the core of the sun and other stars, more realistic models of supernova explosions and better simulations of the structural formation in the early universe.
“Last but not least is that we will be able to build neutrino telescopes, which can be used to observe objects that are more distant than classic telescopes can see,” she says.
---------------------------------
Read this story in Danish at videnskab.dk
Translated by: Michael de Laine