Researchers' Zone:
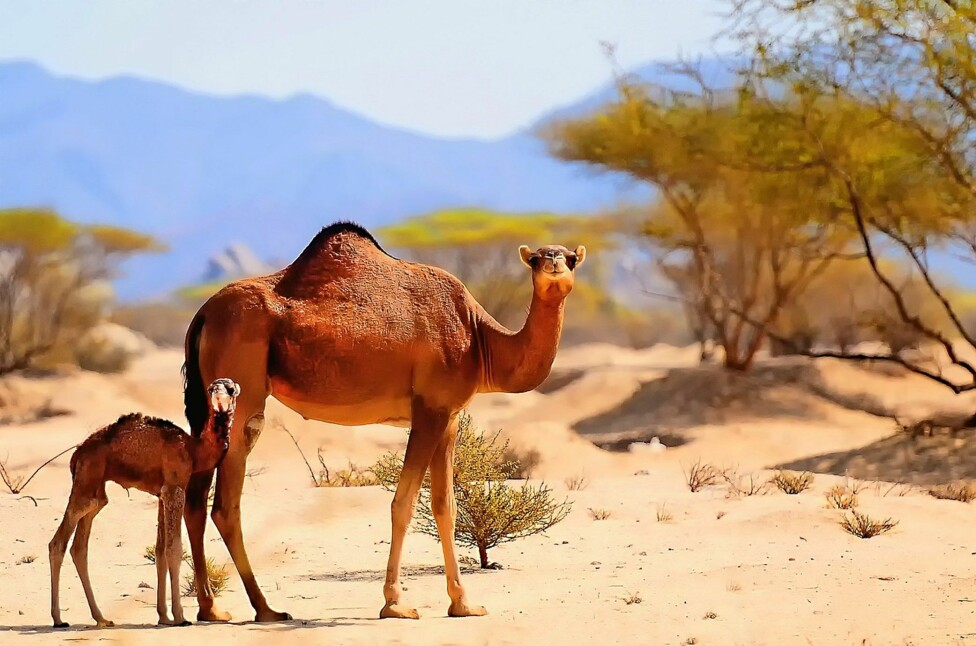
Nanobodies – a smaller and better version of antibodies?
Nanobodies, a protein derived from the camel’s immune system, can prove very helpful in combating a variety of diseases and ailments. But what are they exactly and how far has the current nanobody research come?
Scientists always endeavor to discover new ways to treat anything and everything. Surprisingly, a promising tool in this pursuit has been discovered in a rather peculiar place; the immune system of camels.
Unlike the rest of us mammals, camelids (such as camels and llamas) do not solely produce regular antibodies against foreign threats, but additionally have a quite interesting protein – the nanobody – that could be very useful for us humans as well.
Last year, the first nanobody drug was approved to treat a rare blood disease. This drug may be the first of many to come as research groups all over the world are experimenting with nanobodies as a mean to combat diseases ranging from Alzheimer’s to snakebites, cancer and HIV infections.
However, before getting into the potential use of nanobodies, one need to take a look at what they are and how our immune system works.
What are antibodies?
Every day, our immune system fights an endless battle against foreign and domestic threats, such as bacteria or viruses. The immune system produces a plethora of different components, each with a specific role to recognize, recruit help, or destroy hazards inside of our bodies.
One of the most essential tools in our immune system’s arsenal are antibodies. These specialized proteins work as a kind of heat-seeking missile that can recognize and bind to a target called the antigen, thereby ensuring the antigen’s neutralization.
The most common type of antibody found in mammalian blood is called immunoglobulin G, or IgG for short. It is a fairly large, Y-shaped protein that consists of four different peptide chains; two heavy-chains and two light-chains (figure 2A).
Most of the domains in these chains are similar throughout all IgGs, and they are therefore called the constant regions (indicated with blue in figure 2).
These regions are involved in determining the function of the antibody and ensuring that the antibody, upon antigen binding, provides the correct signal to immune cells. This could, for example, be to recruit natural killer cells, to eliminate virus infected cells or to tell the immune cell to destroy and ’eat’ invading bacteria.
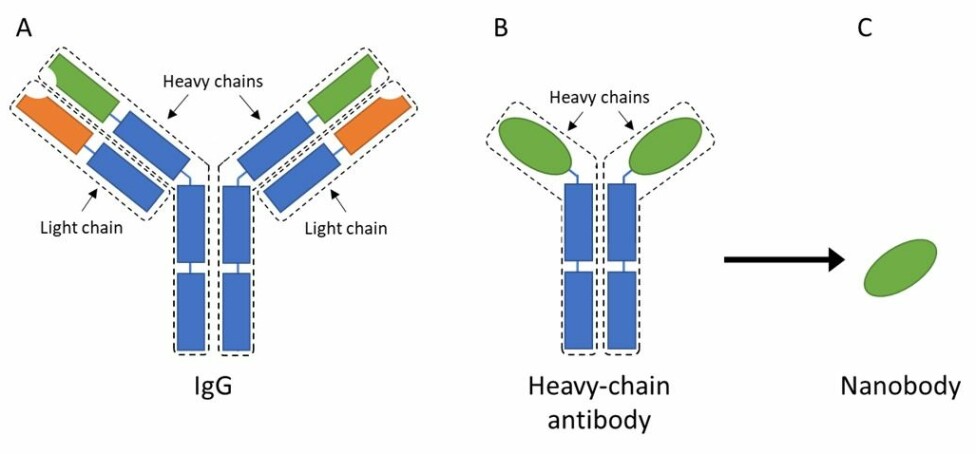
However, the regions that interest most scientists are the variable regions, located at the tip of each arm (indicated with green and orange in figure 2). As the name states, this region can vary and, thus, change the antibodies’ ability to bind to different antigens.
The hidden gem inside the camel
More than 25 years ago, in 1993, a group of scientists discovered that camelids have a different type of antibody in their bloodstream.
These antibodies only contain the heavy-chains, and they were therefore creatively named heavy-chain antibodies (figure 2B). These antibodies maintained the same function as IgGs, as a scout in the immune system that recognize invaders or toxins.
However, to discover such antibodies that lacked the light-chain was surprising, since the light-chain is essential for human and other mammalian antibodies to function.
Scientists took the discovery of heavy-chain antibodies even further by isolating the part that is responsible for antigen binding from these camelid antibodies, thus creating a small antibody fragment that is still able to bind and recognize antigens.
Due to its small size of a few nanometers, this antibody fragment was then dubbed a nanobody (figure 2C).
Size does matter – and sometimes smaller is better
It turns out that nanobodies have several key advantages when compared to IgGs. One of the major advantages of the nanobody lies in its small size – a nanobody is only 1/10 the size of an IgG.
This small size allows the nanobody to penetrate tissue faster and deeper, compared to IgGs. An example where this comes in handy, is when looking for tumors with e.g. a PET scan.
Here, the nanobodies would bind to the tumors, and if a radioactive label is added to the nanobody, it is possible to find and locate tumors. Several pre-clinical trials are being conducted currently using this approach and so far, they have been showing very promising results.
The small size of a nanobody also allows for rapid clearance from the blood stream through the kidneys, which, in this diagnostic context, is highly advantageous, since you don’t want the radioactive labelled nanobody to remain in your body longer than necessary.
However, in many therapeutic settings, the fast clearance can be detrimental, since it limits the nanobodies’ time to bind and neutralize their targets. Nevertheless, via smart biochemistry, nanobodies can easily be engineered to stay in circulation for as long as required.
Increased stability makes nanobodies easier to handle
Another key advantage of nanobodies over IgGs is their increased stability. This is largely linked to their significantly simpler structure (a simple single speed bike is a lot less likely to break than a complex 24 gear one). Hence, it is often much easier to develop and produce nanobodies in the lab than the more complex IgGs.
Finally, the high stability of nanobodies also means that they can potentially be transported and stored without refrigeration, which is particularly useful in rural and remote areas. This, for instance, is highly relevant in the context of snakebite envenoming.
Over 100,000 people die by snakebites each year and the majority occur in remote and impoverished regions in Sub-Saharan Africa and South and Southeast Asia. Current IgG-based treatments are scarce and need to be refrigerated when stored, which further complicates matters.
Nanobodies do not suffer from the same limitations and, thus, could improve the global reach of antibody treatments, even to the most remote areas.
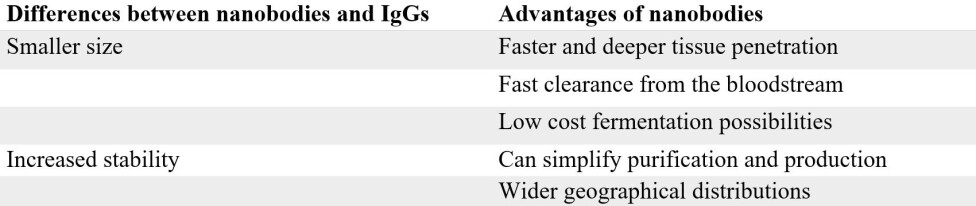
Nanobodies as cancer treatment?
In addition to being useful for immunoimaging to locate cancer, nanobodies can potentially be used to treat cancer via immunotherapy.
IgGs are currently used in immunotherapies, yet, this treatment is only effective in a fraction of cancer patients, and the efficiency is dependent on the location of the cancer and the cell type involved.
However, by exploiting the high tissue penetration rate of nanobodies, it could be possible to target cancer cells that might otherwise be impossible to treat with IgGs. Currently, several clinical trials are being conducted with nanobodies to be used in both immunotherapy and -imaging.
In Brussels (Belgium) they are investigating whether nanobodies can improve PET/CT scans for a variety of different cancer types including breast- and skin cancer and in Zhengzhou (China) nanobodies are being tested as an alternative treatment against relapsed or refractory myeloma and B cell lymphoma.
2019: The first nanobodies arrives on the market
A breakthrough moment for nanobodies was when, in 2019, the FDA (the U.S. Food and Drug Administration) approved the first treatment using nanobodies.
This nanobody-containing drug, called Caplacizumab, is used to treat acquired Thrombotic Thrombocytopenic Purpura (aTTP), a rare, life-threatening blood disease, where the blood platelets clot throughout the body and a breakdown of the red blood cells leads to renal failure, heart damage, and neurological deficits.
Untreated, aTTP will most likely result in coma or death, but even if treated with the previously available treatments, the mortality rate is as high as 20 percent. The company Ablynx discovered that nanobodies could be used as a drug to treat aTTP and developed nanobodies that target a protein found in the blood stream named ‘von Willebrand factor’.
In a healthy person, the von Willebrand factor helps the blood platelets adhere to a wound site to close the wound, but in aTTP this system is in overdrive. As a result, the von Willebrand factor causes the blood platelets to form blood clots everywhere in the blood stream.
Caplacizumab blocks the binding between the von Willebrand factor and the platelets, and thereby prevents the protein from inducing blood clot formation.
Studies show that when using the nanobody-drug, not only is the mortality decreased, but severe aTTP complications, such as major blood clots and strokes, are reduced by 74 percent.
Nanobodies to target diarrhea virus in children?
The approval of Caplacizumab might be the first of many nanobodies that are approved for diagnostics or treatments. A clinical trial with nanobodies targeting the diarrhea-causing rotavirus has also been conducted with promising results.
The rotavirus is one of the leading causes of morbidity and mortality in children under 5 years of age, with up to half a million deaths per year.
Currently there is no treatment or vaccine against rotavirus, so a treatment or cure with e.g. nanobodies could potentially save hundreds of thousands of children each year.
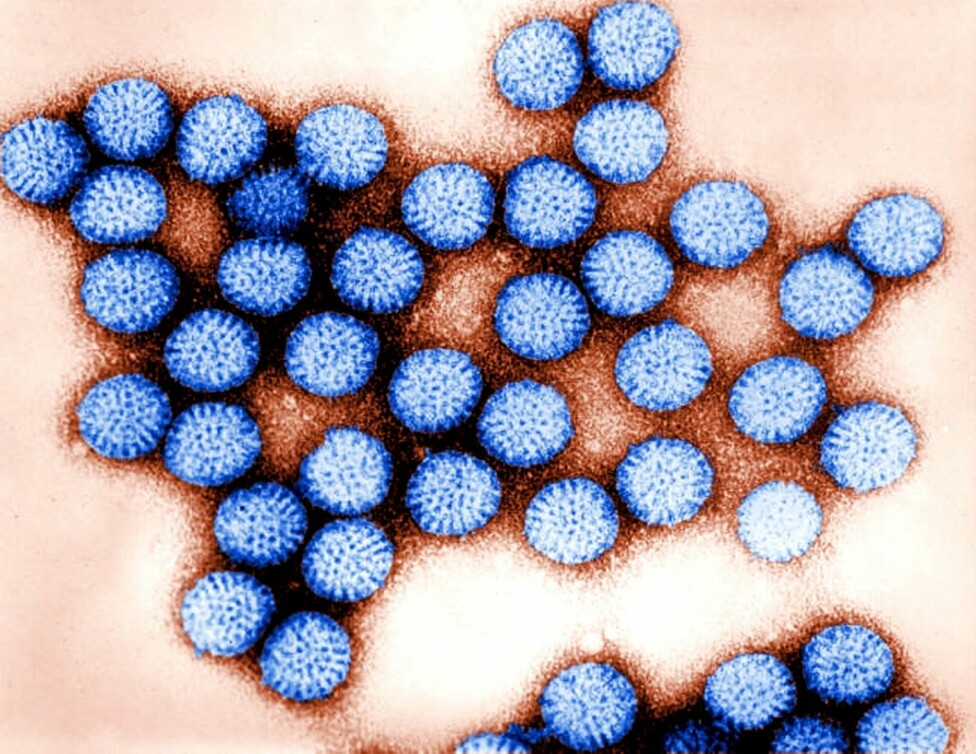
The nanobody can neutralize paralyzing toxin
Supplemental to nanobodies ability to treat infections and other diseases, nanobodies have also shown great potential in the treatment of venomous bites from e.g. snakes, spiders, and scorpions.
A nanobody that has been isolated has the ability to bind and neutralize the highly lethal α-cobratoxin, the main component in the monocled cobra’s venom.
When bitten by this cobra, the α-cobratoxin travels through the body and on its way, the toxin blocks the signals sent from the brain and the muscles. The brain is left incapacitated and as a result the body is paralysed.
The nanobody works by binding to the toxin, and, thus, preventing the toxin from blocking signalling from the brain or muscles, and the nanobody will keep this binding until the toxin is destroyed.
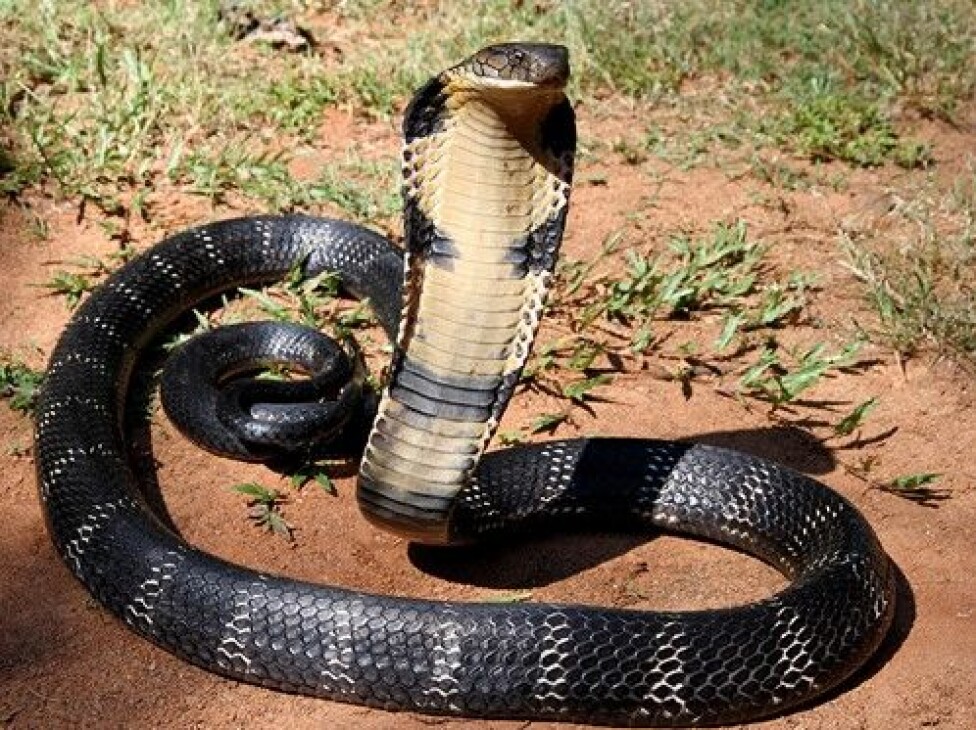
The key to discover novel and innovative possibilities
The examples presented here are just a few of the many exciting examples that scientists are applying nanobodies to. Other examples include research into possible treatments against central nervous diseases, such as Alzheimer’s and Parkinson’s.
Presenting a comprehensive summary of all exciting developments in the field unfortunately falls outside the scope of this article.
The take-home message is that the many of the advantageous features of nanobodies, including the small size and increased stability, allow scientists to discover these novel and innovative application possibilities.
And with the first nanobody drug already out on the market, it seems like it is only a matter of time before we see the next one available.
Disclosure statement: Andreas Hougaard Laustsen is a founder and shareholder in the company, Bactolife ApS, which employs nanobody technology. No examples of work from this company are presented in the text. The authors declare no other conflicts of interest.
Read the Danish version at Videnskab.dk's Forskerzonen.
References
Anna Damsbo Jensen’s profile (DTU)
Timothy Patrick Jenkins’ profile (DTU)
Thomas Fryer’s profile (Cambridge)
Andreas Hougaard Laustsen’s profile (DTU)
'Toxin Neutralization Using Alternative Binding Proteins', Toxins (2019), DOI: 10.3390/toxins11010053
'Nanobodies: Natural Single-Domain Antibodies', Annual Review of Biochemistry (2013), DOI: 10.1146/annurev-biochem-063011-092449