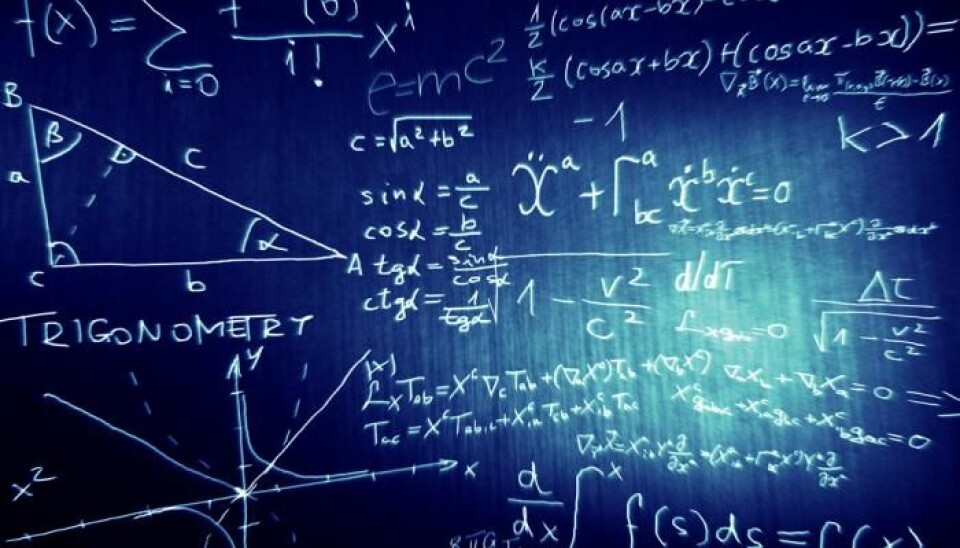
Can large objects exist in a quantum state?
Quantum physics is the study of objects at the tiniest atomic level, but new research will investigate whether quantum technology can be used on a large scale.
Even after an acclimatisation period of more than 100 years, quantum mechanical phenomena still conflicts with our intuition of how nature works. Quantum mechanics confuses and marvels us in equal measure.
We have long known that particles can be in a superposition—both here and there at the same time. We can measure this in the laboratory. But does this also hold true for larger objects? That is the question.
Many people are comforted by the fact that the bizarre phenomenon of Schrödinger’s Cat, which is said to be both dead and alive at the same time, belongs to the atomic world, far removed from our daily lives.
But for scientists this is no comfort—but a challenge!
Can it really be that quantum phenomena are limited to the microscopic world? And if so, what prevents larger objects from existing in superposition?
This question is the focal point of a new research project in the physics department at the Technical University of Denmark (DTU). If the project is successful, it will not only be another victory for the theory of quantum mechanics—it will force us to change our understanding of the world and our view of what is and is not possible.
Read More: Physicists want to recruit more young quantum physicists
Is the cat dead or alive?
Imagine a cat trapped inside a box with a vial of poisonous gas, a radioactive source, and a Geiger counter. When the Geiger counter registers radioactive decay, the vial shatters, and the cat is killed by the gas. From the outside, we cannot directly observe the cat, but we know that after a certain amount of time there is a 50 per cent probability of the decay having occurred. At this point, how is the cat doing?
Quantum mechanics says that the cat is in a superposition: alive and dead. At first sight, we might accept that description, but it is important to note that superposition does not mean that there is a fifty-fifty chance that the cat is either alive or dead, and that we simply do not know which outcome is true. It’s not like flipping a coin and choosing heads or tails without knowing the final result.
What quantum mechanics says is that the cat is both dead and alive—at the same time. That is the most typical interpretation. A more correct formulation is probably that the cat simply does not have a well-defined state in classical physics and that there is no answer to the question “is the cat dead or alive?” until we open the box and take a look.
The situation is just as paradoxical and contrary to classical physics, if the experiment involves the cat being replaced with a simple microscopic atom. The question then becomes whether the atom is at one location or another.
Erwin Schrödinger’s thought experiment was designed to show the absurdities of quantum mechanics. By using a large, living object, he undoubtedly made it even more spectacular and titillating and it remains so to this day!
Can large objects really be in two places at once? Experiments conducted the world over speak in their own language: Physical objects can assume contrary properties, so long as we don’t observe one or the other. We do not experience it in our everyday lives because large objects are constantly observed, either directly or indirectly via the influences from their surroundings.
Read More: Hunting for the “quantum limit”
A scientific breakthrough
How big can an object be and still exhibit quantum properties? And which mechanisms enforce this limit?
These are open questions and the possible answers are highly technical.
Schrödinger’s cat states have so far only been observed for electromagnetic fields and individual atoms. But the almost explosive development of optomechanical research (the interaction of light and mechanical objects), holds great promise that quantum mechanical superposition might be achieved on a larger scale.
This would be a scientific breakthrough!
Most revealingly, it will allow us to test why we don’t experience superposition in daily life. That is, the physical mechanisms responsible for why we so far have only observed superposition in small particles in the laboratory and not in larger objects.
Read More: Scientists move closer to developing a quantum computer
To do this we test a number of decoherence models. Coherence means that two objects are in a fixed relation to each other. Decoherence is the gradual loss of such relations, for example, the transition from the cat being dead and alive to being dead or alive.
We hope these tests will cast light over the apparent limits between the quantum world and our “classical” world.
We need to be careful with our choice of words here. Though the transition between quantum mechanics and classical mechanics is traditionally termed as ‘the quantum-classical boundary,’ separating the realms of classical and quantum mechanics, it is hard to believe that there is a hard dividing line between the two physical regimes.
This is where it gets a bit technical, but a more realistic physical model will be that one or more decoherence mechanisms (e.g. gravity) gradually increases in strength as objects become bigger, which leads to a collapse of the superposition into one particular state within a spectrum of well-defined classical states.
In other words: The cat is forced into one state or the other.
Read More: Gamers solve problems faster than a computer
A bigger Schrödinger’s cat
In 2016, our research group published a theoretical study in Physical Review Letters that showed you could exploit optomechanical interactions to bring a vibrating mechanical object into a Schrödinger’s cat-like state.
Imagine a membrane of a speaker. As it oscillates, it changes the density of the molecules and creates sound waves. If we turn the process around so that we transmit sound waves onto the membrane, the flow of over pressure and under pressure caused by the sound wave will push and pull on the membrane and cause it to move.
But how will the membrane react if it is exposed to under pressure and over pressure simultaneously? This is what we will do in the experiment, but with light and a tiny mechanical object. The result is that the membrane ends up in a superimposed condition of two opposite oscillation phases—a macroscopic superposition where it is both in one phase and the other, simultaneously.
It might be difficult enough to create a theoretical picture of the process, but the practical realisation is unfortunately even more challenging.
One way is to transfer a Schrödinger cat state of light to a mechanical object. That means that laser light, which simultaneously has two different levels of brightness, is transferred to the mechanical object. In this way, what originated as a superposition of the two levels of brightness will end as a superposition of two different mechanical deflections.
Read More: Scientists need your help to build a quantum computer
A condition for this to happen is that the interaction between light and mechanics is so short that the mechanical movement is effectively frozen and stands still as the interaction takes place.
Under those circumstances, the mechanical oscillations are simultaneously effected by the optical cat’s two different brightness and is pushed simultaneously to different positions in its pattern of oscillations.
For the study to succeed, a number of particular conditions need to be met:
- First and foremost Schrödinger's Cat states of light must be generated.
- The optomechanical interactions should be precisely timed in relation to the mechanical oscillations.
- The mechanical oscillator should be of high quality for it to give time to both generate and verify superposition before the oscillation dies out.
The new research project will tackle these challenges head-on using tiny trampoline-like mechanical objects. They are made of silicon nitride (Si3N4) and consist of a central quadratic membrane measuring 100 microns on each side, connected to a fixed frame with four tethers, which are five microns wide and just 50 nanometres thick.
The tethers provide the springs to the trampoline, which allows the membrane to swing up and down with a frequency of around 160 kiloHertz— somewhat faster than a regular trampoline in your garden.
Read More: The nanomedicines of the future will build on quantum chemistry
Requires extremely controlled conditions
Production of mechanical objects on a micro- or nanoscale requires the same high tech manufacturing processes as those used by the computer industry to produce microprocessors. Luckily, at DTU we have access to such facilities and world class manufacturing equipment via DTU Danchip.
In theory, the trampolines are simple to make. But in practice it requires extremely controlled conditions to create nanoscale products with the desired properties.
The process begins with a layer of silicon nitride with the desired thickness being deposited on a silicon wafer—a thin sheet of silicon similar to those used in the production of microchips. It has a very high material tension, which is required to make a high quality oscillator. This results in a slow dampening of the mechanical oscillations, which means that we can measure and verify the creation of a superposition before the oscillations fade away.
The trampoline structure is printed lithographically on one side of the wafer with ultraviolet light, producing a shadow outline of the trampoline using a photoresist—a light sensitive material. A chemical transformation occurs on the illuminated side of the photoresist and leaves only the unexposed part behind.
We then remove the nitride not protected by the overlaying photoresist, revealing the trampoline structure. Finally we release the trampoline by etching all the way through the silicon wafer.
Read More: Scientists get to grips with a tricky liquid
Has to be kept cold!
From a quantum physicist’s viewpoint, nothing is ever completely still or at rest. Even in the quantum mechanical ground state there is always quantum noise (so-called zero point fluctuations), as required by Heisenberg’s Uncertainty Principle.
This also applies to mechanical objects like the trampolines. Their quantum noise equates to oscillations the size of the diameter of a single atom and are extremely difficult to observe.
To observe them requires fighting through a thick layer of thermal noise caused by the constant bombardment of particles from the surroundings.
The higher the temperature of the surroundings, the more powerful the thermal noise in the mechanics. This needs to be overcome in order to achieve superposition of a macroscopic object.
The object first needs to be brought to its ground state. To achieve this, our experiment will have to take place inside a cryostat—a device that creates extremely low temperatures and completely isolates the experiment from the surroundings. The temperature will be as low as a few thousandths of a degree above absolute zero (-273.15 degrees Centigrade).
Even at such low temperatures, our trampoline oscillator will still be excited by tens of thousands of vibrations—so-called phonons, the smallest unit of vibration. In the quantum ground state, there is well under one phonon on average.
Weighing just one nanogram, the trampoline is still tiny, but large enough to be seen with the naked eye and in that sense it can be considered as being macroscopic.
Read More: New satellite will test Einstein’s theory
“No pain, no gain”
For a quantum physicist, there’s not much that beats the prospect of studying some of the most fundamental aspects of quantum mechanics. Equally, it is one of the more risky projects you can do.
We undeniably have a number of technical challenges and any number of unforeseen problems ahead of us. But by working through the process of solving these, we will obtain worthwhile new knowledge and skills to tame the quantum world.
This is basic research but the collected outcomes will also be relevant for our other projects, developing quantum technologies.
So despite the hard work ahead, we’re looking forward to it, and all the grey hairs it may bring.
---------------
Read this article in Danish at ForskerZonen, part of Videnskab.dk
The project is funded by Villum Fonden and will be continued with funding provided by the Danish National Research Foundation to the Center for Macroscopic Quantum States, led by Professor Ulrik L Andersen, DTU Physics.
Translated by: Catherine Jex