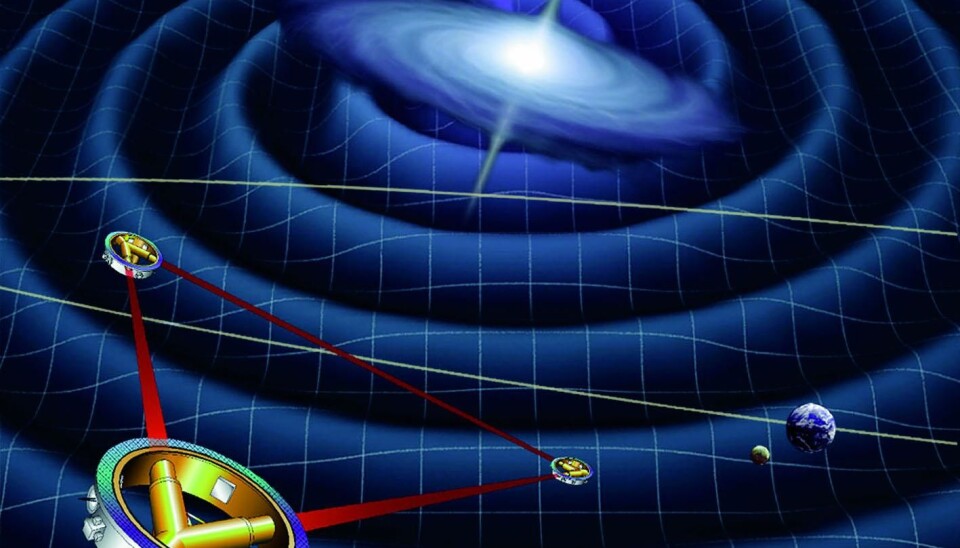
Big Bang mission relies on Danish technology
Danish researchers will supply crucial technology for a new mission to find evidence for the Big Bang theory. The mission requires technology that can measure distances of five million kilometres with the precision of a picometre – that's 0,00000000001cm.
Almost a century ago, Albert Einstein theorised that the universe bends around stars and planets.
The theory could explain natural phenomena such as tiny gravitational variations, which could be observed differently in outer space than on Earth.
Einstein's theory also included the existence of gravitational waves, which can be explained as ripples in the universe – a bit like setting a bowl of blancmange into motion.
Too small to prove
These waves are remnants of intense astronomical events such as colliding stars and merging black holes.
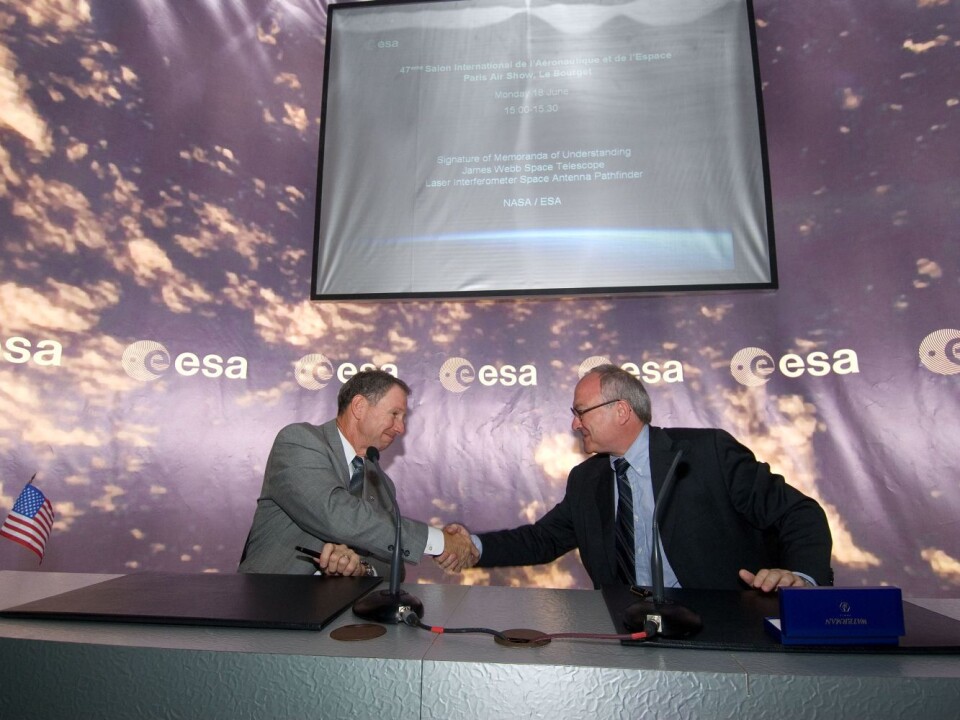
Great as his theory was, Einstein never managed to find physical evidence for his theory about gravitational waves.
They were simply too tiny to be measured with contemporary technology. Nevertheless, these waves form the basis of hypotheses such as the Big Bang and the origin of black holes.
Danish researchers help LISA
The European Space Agency (ESA) has now established a new space mission, known as LISA, in its search for the physical evidence that Einstein failed to find. And Danish scientists from the National Space Institute at Denmark's Technical University (DTU Space) are at the forefront of the preparations.
In collaboration with the German Albert Einstein Institute and Danish electronics manufacturer Axcon, DTU Space is developing a laser gauge that could measure these tiny variations.
“It's a small yet central part of the project,” says senior researcher Søren Brandt, of DTU Space. “Our task is to show that we can measure huge distances in space with the required precision.”
Laser registers gravitational waves
The LISA mission consists of sending out three satellites in orbit around the sun, creating an isosceles triangle.
The length of each leg of the triangle will be five million kilometres. This distance must remain constant at all times because when a gravitational wave passes through the solar system, it can displace the measuring devices inside one of the satellites, causing a slight displacement in relation to the other two satellites.
Variation of 0.00000000001cm
DTU's new laser technology sets out to solve that problem. With its extreme accuracy, it not only aims to prove the existence of these waves, but will also measure their direction and speed. The gravitational variations are so miniscule that they are measured on a so-called picometre scale. A picometre corresponds to 0.00000000001cm.
Counting light waves
To measure distances, astronomers use laser beams to do what is known in popular terms as counting light waves.
Laser light waves have a wavelength of 500 nanometres (a nanometre is 1/100 of a picometre), so it takes a few of those when you're measuring distances of five million kilometres.
In order to maintain an accurate distance at picometre levels, you not only need to identify the various phases (from the top to the bottom of the light wave) over the five million kilometres; the measuring devices must also be able to determine when a phase gets a bit off the line when a gravitational wave passes by.
Terrestrial interferences do not exist in outer space
No-one has so far managed to construct a sufficiently sensitive measuring device, partly because such a device would also be challenged by terrestrial interferences. A distant earthquake, the ventilation system in the laboratory or a truck on the road outside could be more than enough to muddle up the measurements.
These problems, however, would not occur out in space. Although a satellite could be disrupted, for instance by solar wind, the measuring device itself is locked inside a cube which floats around in a state of weightlessness inside the satellite.
“The satellite itself can easily be affected by solar wind,” explains Brandt. “But small engines make sure that the satellite adjusts itself when it's being pushed. That way, the cube on which the variations are measured will be protected from external forces.”
Knowledge moves us forward
Why do we need to know about gravitational waves when Newton's law of gravitation works just fine?
ScienceNordic asked Allan Hornstrup, the head of the department of astrophysics at DTU Space:
“We don't know what results we'll get from measuring gravitational waves. But it's a prerequisite for basic research. Only by understanding the laws of physics can we start looking at what use we can make of them.”
This point has been proven time and time again in science. One example is Danish physicist Niels Bohr's quantum theory, which changed our conception of how electricity works.
It wasn't until Bohr's model of how electrons switch places had been proved that this knowledge could be used to invent the semiconductor, which has formed the basis for all our modern internet and mobile phone communication.
“It's really exciting to be part of research that in principle could revolutionise the way we understand the laws of physics, and which could lead to completely unforeseen inventions,” says Hornstrup.
Closer to the origin of the universe
If the mission succeeds in measuring gravitational waves, it would provide us with a new tool to 'see' phenomena that we have no way of accessing today.
Light can give us a great deal of information. It makes it possible, for instance, to figure out which elements the various stars are made of. This is done by letting the light from the star pass by a prism and then analysing the colour spectra that come out of it.
If successful, the mission can teach us a lot about the origin of our galaxy, since it will enable scientists to read off the cosmic microwave radiations, which is the afterglow of the Big Bang.
In the early years following the Big Bang, the universe was hazy and opaque. The light waves were disrupted by free electrons, which is why they cannot be measured.
This explains why the first 380,000 years of the formation of our universe have remained a mystery.
But gravitational waves could pass without being affected in the same way as light waves were. This means that if the mission succeeds in measuring gravitational waves, it is theoretically possible to determine what happened in the very first seconds after the Big Bang.
Read the article in Danish at videnskab.dk
Translated by: Dann Vinther
External links
- Animated video about the LISA mission
- About LISA on the European Space Agency's website
- DTU Space website
- The Albert Einstein Institute website